
Imagine a world without electrical resistance. A world with powerful wind farms in North Dakota and perfectly positioned solar farms in Nevada. A world where nuclear power plants are safe, efficient and aren’t an eyesore. Imagine all of this clean energy being generated and then flowing to the world’s population centers without any energy loss.
That’s the world promised by superconductors, materials that conduct electricity with no resistance and expel a magnetic field.
“Right now, power transmission lines have transmission losses of around 10% to 20%,” said Inna Vishik, an associate professor in the Department of Physics and Astronomy. “If those losses could be eliminated, that could translate into cost savings or greater energy abundance.”
While this world of lossless transmission lines is currently a blue-sky idea, progress is being made towards making that world a reality. But the path to get there is one dappled with sometimes farfetched claims.
Usually, superconductivity is a low-temperature phenomenon. But every so often, reports of high-temperature or room-temperature superconductors circulate through the scientific community.
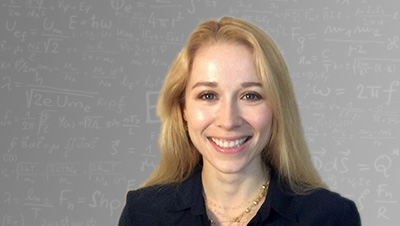
“We even have a term for these,” Vishik said. “They’re called unidentified superconducting objects, or USOs, and they show up pretty regularly every couple of years or so."
With the advent of social media, many of these purported room-temperature superconductors go viral, leading to a plethora of news headlines.
During her recent Dean’s Faculty Fellow Public Talk, Vishik guided attendees through “Superconductivity: The Hype and the Reality.” The lecture took a sobering look at the field while also providing insight into the latest research developments from Vishik and her UC Davis colleagues.
The Hype
The summer of 2023 was the summer of LK-99. In July of that year, a research team from South Korea posted a paper on arXiv.org — an open-access, pre-print website — reporting that a material they called LK-99 could carry electricity at room temperatures with zero resistance.
“It took off on social media,” Vishik said. “And it took off in print media. As different publications explored investment opportunities, there was an element of some DIY citizen science to try to replicate the results.”
“It really took the world by storm for a couple of weeks,” she added.
The hype died down when multiple research teams failed to replicate the results. Scientific sleuths further investigated LK-99, a compound composed of copper, lead, phosphorous and oxygen.
Rather than being a legitimate superconductor, “studies have shown that impurities in the material — in particular, copper sulfide — were responsible for sharp drops in its electrical resistivity and a display of partial levitation over a magnet, properties similar to those exhibited by superconductors,” read a report in Nature.
Copper sulfide “undergoes a phase transition at 100 degrees Celsius, exactly around the temperature that LK-99 was reported to superconduct,” Vishik said. “And especially when it’s exposed to oxygen, this phase transition is accompanied by a large change in resistivity.”
Copper sulfide “is actually a very interesting material, but it’s not a new material,” Vishik added. “It’s studied for other energy applications, but it’s not a superconductor.”
The Reality
Superconductors capture the imagination of the public due to their potential technological applications. Vishik’s intrigue, and perhaps the curiosity of other physicists, stems from the paradoxical properties these materials embody.
Take electrons, for example. Typically, these negatively charged particles repel one another. But in a superconductor, electrons bind together to form cooper pairs. Usually, quantum mechanics is reserved for microscopic particles. But superconductors eschew this rule.
“A superconductor is a macroscopic quantum state,” Vishik said. “So you can have quantum mechanics, not on atomic length scales, but on very large macroscopic length.”
According to Vishik, the most promising family of superconductors are cuprate superconductors, which are fashioned from layers of copper and oxygen, and often, other metal oxides. First discovered in 1986, cuprate superconductors, like LK-99, had a lot of media hype. TIME Magazine even dedicated a 1987 cover story to them.
“They promised a superconductivity revolution with cars that plug into walls,” Vishik said. “We got that, but they’re not superconducting cars.”
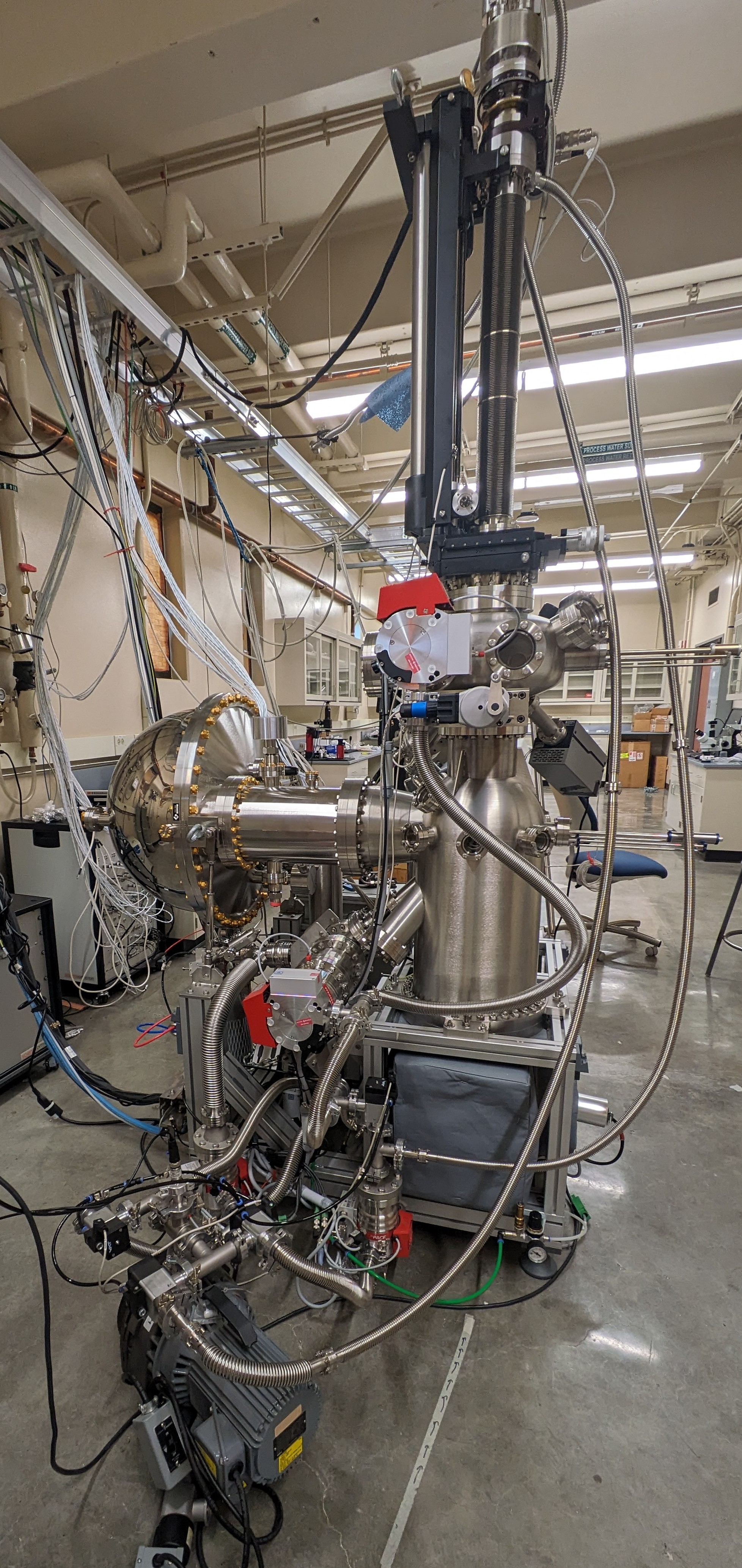
It’s only been within the last handful of years that cuprate superconductors have found viable applications, including in the burgeoning nuclear fusion economy, according to Vishik,
“That’s to say, if a room temperature superconductor is discovered tomorrow, it would very likely be several decades before it is actually fully deployed,” Vishik said. “If we want one in the next decade, cuprate superconductors are the only show in town.”
In her lab, Vishik and her colleagues employ angle-resolved photoemission spectroscopy, among other techniques, to reveal the electronic structure and molecular dynamics of superconducting and quantum materials. The funding from her Dean’s Faculty Fellowship is furthering her work to elucidate the electronic fingerprints of superconductors. This is accomplished via the photoelectric effect, which is when light particles (photons) hit a material with so much energy that electrons are emitted from that material.
“The electron that’s ejected, it’s still carrying information about how it was moving back inside the material,” Vishik said. “We measure the energy of those emitted electrons, we measure the emission angle and from that, we’re able to back out the energy and momentum that they had back inside the crystal.”
Vishik not only does this in her lab on campus, but she and her team also use synchrotrons at nearby National Laboratories.
“Once this electronic fingerprint is measured, we can discuss what [the material] is, why does it have these properties and then how will it behave in a device or in a circuit,” Vishik said.
Learn more about Vishik’s research on her lab website.